Rathish Rajan
Simo Huhtanen
Juha Junkala
Tuomo Viitala
Antti Takalo
Tero Köyhäjoki
Egidija Rainosalo
Strong biomass availability, the success of the existing forest industry, and the development of new products are the most important factors contributing to the development of the bio–economy. Utilizing natural fibers in polymer composites can considerably reduce the usage of the polymer and as result usage of oil resources. Renewable and abundant wood-based fillers are used in the production of synthetic or bio-based polymers matrix composites (WPC). The applications of WPC can be expanded to new industries by tailoring properties. In this study, the electrically and thermally conductive wood plastic composites were developed.
Biocomposites play an essential role in various industries being an alternative to materials from fossil-fuel (Alemdar & Sain, 2008). Biocomposites are produced by embedding natural fibers of the plant or animal origin in a bio-based or synthetic polymer matrix (Sapuan, 2017). Wood-plastic composites (WPC) are biocomposites produced from wood fiber and synthetic or bio-based plastics (Figure 1). Commercially available WPC is dominated by wood-filled thermoplastics such as polypropylene (In Europe) and high-density polyethylene (In USA) (Partanen, 2020). Currently, WPC is used in building & construction, automotive, and household applications.
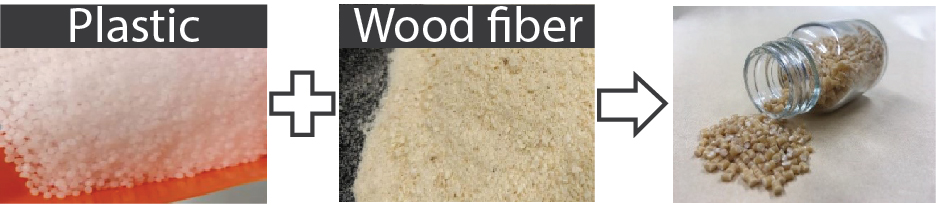
Strong biomass availability, the success of the existing forest industry, and the development of new products are the most important factors contributing to the development of the bio–economy. The applications of WPC can be expanded to new industries by tailoring the properties of wood-plastic composites. As an example, incorporating electrical and thermal conductivity in WPC can open its application in products demanding these functional properties. Conductive (electrical and/or thermal) thermoplastics are available in the market which is used in producing products requiring anti-static properties, electromagnetic interference shielding (EMI shielding) PILKKU and for those products used in thermal management in the electrical and electronic industry (Figure 2) (Biron, 2018).
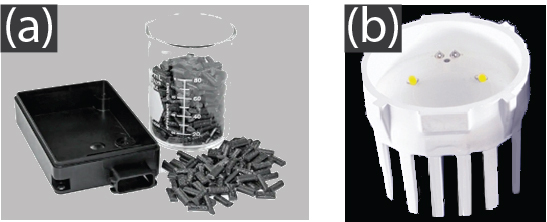
Aim of the study
The main aim of the study was to develop a new generation of biocomposites consisting of functionalized WPC for construction, energy, electrical and electronic industry. Electrically and thermally conductive fillers were incorporated into wood plastic composites and its effect on various properties of WPC was studied. Another objective of the research was to develop a method for robot–assisted 3D printing from conductive WPC granules. (Figure 3.)
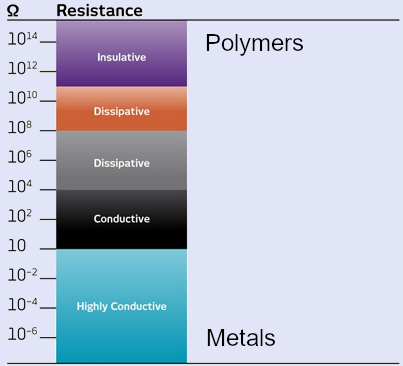
Development of thermal and electrically conductive WPC
All the composites developed in this study were based on polypropylene. The conductive WPC granules were produced by melt mixing polypropylene, wood fiber and respective conductive fillers in a co-rotating twin-screw extruder. Test specimens were produced either by hot pressing the conductive WPC granules or by injection molding method. EMI-shielding enclosures were produced by direct 3D-printing from produced conductive WPC granules (Figure 4).
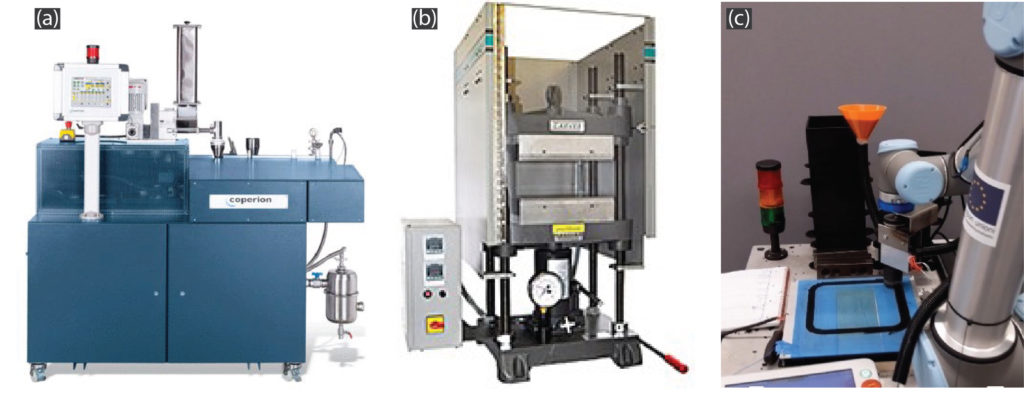
Conductive WPC prepared from 60 wt% PP/carbon black masterbatch (MB) and 20 wt% of wood fiber exhibited the highest EMI shielding among all the electrically conductive composites produced in this study (20 dB measured in 100 MHz – 1500 MHz frequency range) (Figure 6). Also, dissipative/anti-static WPC exhibiting electrical resistivity within a range of 104 – 106 Ω.cm was developed. Increasing the amount of wood fiber at a constant carbon black content in composites seems to have an effect in decreasing the electrical resistivity compared to the composite without any wood fiber. This indicates that by increasing the wood fiber content, better distribution of carbon black is achieved. Improved distribution of carbon black has formed more conductive path through which the resistivity decreased. (Figure 5)
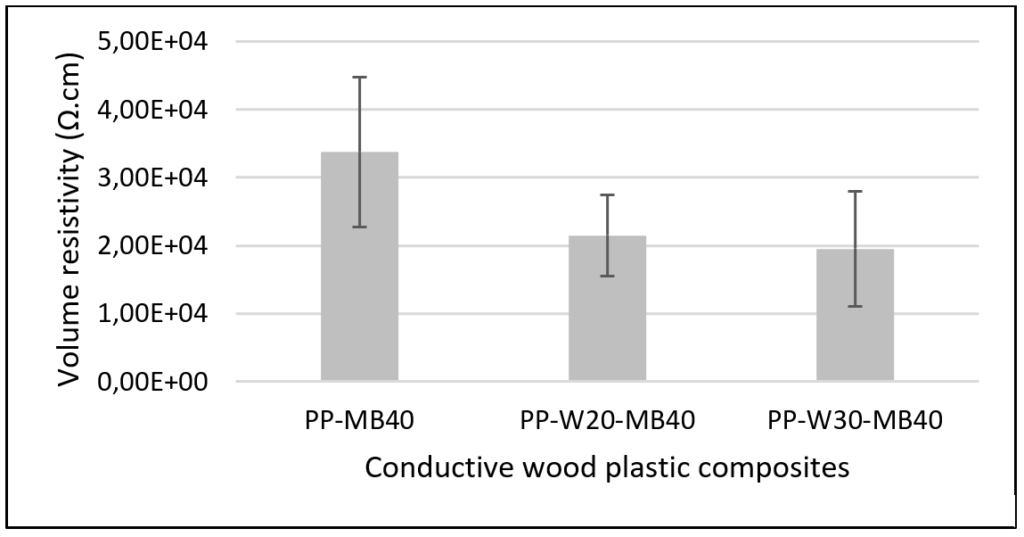
Graphene nanoplatelets (GNP) as electrically conductive fillers in composites were also developed in this research. It was found that the lowest resistivity (106Ω.cm) was reached with a graphene content of 15 wt%. Considering the cost of graphene, it was found that carbon black is a cost-effective filler for producing electrically conductive WPC. In general, the electrical percolation threshold is reached with lower GNP loading in composites produced though solution mixing or in-situ polymerization methods (Li, Zhu, Wei, Ryu, Wang, Sun & Guo, 2011). Whereas, the percolation threshold is reached only at a GNP loading of 8 wt% – 15 wt% for composites produced using conventional melt processing method (Jun, Um, Jiang, Lui & Yu, 2018; Li & Zhong, 2011). More studies are needed to develop electrically conductive WPC with lower GNP content by using the conventional melt processing method. Since there is a concern about water absorption in composites containing hydrophilic wood fibres, water absorption studies were done. The 24 hours of water absorption for WPC with 20 wt% of wood and carbon black was found to be approx. 0.40 %, which is similar to the water absorption values reported for commercial WPCs (Wood-Plastic Composites, 2005).
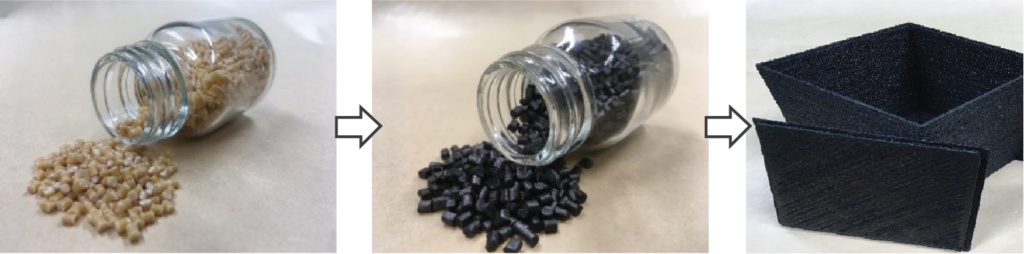
Thermal conductive WPC was produced by incorporating boron nitride (BN), copper, and anthracite (TT). Among all the thermal conductive composites developed in this study, the WPC containing 20 % wood fiber and anthracite (TT) fillers showed the highest through-plane thermal conductivity (5.1 W/m. K). The result shows that the developed electrically conductive WPC can be used in developing EMI-SE enclosures or for producing ESD safe products and thermal conductive WPC can be used in producing products utilized in thermal management such as heat sink materials. (Figure 7)
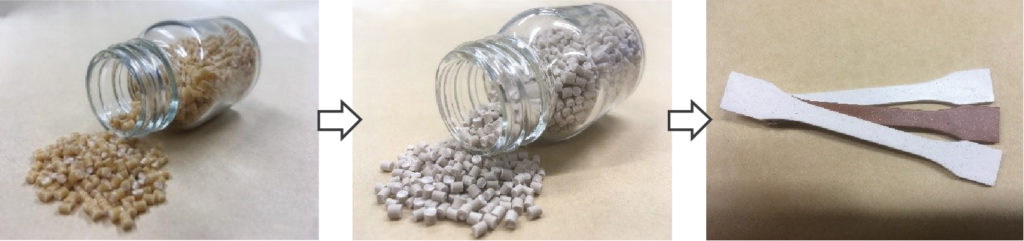
Dissemination and industrial co-operation
The overall findings from this research were shared with SME’s, industries and research institutes especially in the northern region through seminar “Recent advances in functionalized plastic materials, bio-based materials and additive manufacturing” organized in Kokkola on 11.9.2019. The seminar had 53 participants from 12 different companies and 5 universities/ research institutes. The developments from this study were shared and new research collaborations were created by attending events like JEC world 2019 and other seminars where authors attended. The results from the study will be shared also in terms of three publications, among which one is already communicated for publication in a peer–reviewed scientific journal. This study was completed with the association of Priotec Oy, Innomost Oy, Premix Oy, MMI Company Oy, Tactotek Oy, and PolyOne Europe.
Conclusions and future outlook
This study successfully developed thermal and electrically conductive wood plastic composite (WPC). The electrical resistivity of developed WPCs is in range of 104 Ω.cm – 106 Ω.cm. The through–plane thermal conductivity of developed composites was between 1.3 W/m.K – 5.1 W/m.K. These conductive WPCs can be used in developing EMI shielding enclosures, in ESD safe products, and in thermal management of electrical and electronic components. As the research developments on conductive WPC is in the initial stage, the results from this study should act as a point of reference for further development in this topic. The promising results from this study will lead towards developing new sustainable solutions, especially for the conductive plastic market and help in designing new thermal or electrically conductive products for the wood plastic composite industry.
Acknowledgments
The authors are thankful for the grant received to the Smart-WPC project from Interreg Nord program 2014–2020, Region Norrbotten in Sweden and Regional Council of Lapland in Finland.
References:
Alemdar, A. & Sain, M., 2008. Biocomposites from wheat straw nanofibers: Morphology, thermal and mechanical properties. Composites Science and Technology, Vol. 68, Iss. 2, pp. 557-565. Available: http://www.sciencedirect.com/science/article/pii/S0266353807002473.
Biron, M., 2018. Chapter 7 – Plastics Solutions for Practical Problems, in: M. Biron (ed.), Thermoplastics and Thermoplastic Composites (Third Edition), William Andrew Publishing, pp. 883-1038.
Coolics Coolics, heat sink modules. Online document. Available: https://jarikanervisto.kotisivukone.com/standard-coolics-heatsink-modules. Accessed: 13.2.2020.
Jun, Y., Um, J. G., Jiang, G., Lui, G. & Yu, A., 2018. Ultra-large sized graphene nano-platelets (GnPs) incorporated polypropylene (PP)/GnPs composites engineered by melt compounding and its thermal, mechanical, and electrical properties. Composites Part B: Engineering, Vol. 133, pp. 218-225. Available: http://www.sciencedirect.com/science/article/pii/S1359836816321758.
Li, B. & Zhong, W., 2011. Review on polymer/graphite nanoplatelet nanocomposites, Journal of Materials Science, Vol. 46, Iss. 17, pp. 5595-5614. Available: https://doi.org/10.1007/s10853-011-5572-y.
Li, Y., Zhu, J., Wei, S., Ryu, J., Wang, Q., Sun, L. & Guo, Z., 2011. Poly(propylene) Nanocomposites Containing Various Carbon Nanostructures. Macromolecular Chemistry and Physics, Vol. 212, Iss. 22. pp. 2429-2438. Available: https://onlinelibrary.wiley.com/doi/abs/10.1002/macp.201100364.
Sapuan, S. M., 2017. Chapter 6 – Materials Selection for Composites: Concurrent Engineering Perspective. In: S.M. Sapuan (ed.), Composite Materials, Butterworth-Heinemann, Boston, pp. 209-271.
Parker Chomerics, PREMIER PBT Conductive Plastic Pellets. Online document. Available: https://ph.parker.com/us/17051/en/premier-pbt-conductive-plastic-pellets. Accessed: 13.2.2020.
Partanen, A., 2020. Natural fibre-reinforced plastics: establishment and growth in niche markets. Available: http://news.bio-based.eu/natural-fibre-reinforced-plastics-establishment-and-growth-in-niche-markets/. Accessed: 12.2.2020.
Premix group Electrically conductive and static dissipative compounds. Online document. Available: https://www.premixgroup.com/electrically-conductive-plastics/pre-elec-product-family/. Accessed: 13.2.2020.
Wood-Plastic Composites: Weathering Quality Issues, Plastics Technology Magazine, Sept 1, 2005. Online document. Available: https://www.ptonline.com/articles/wood-plastic-composites-weathering-quality-issues. Accessed: 13.2.2020.
Rathish Rajan
RD expert
Centria University of Applied Sciences
Simo Huhtanen
RD developer
Centria University of Applied Sciences
Juha Junkala
project engineer
Centria University of Applied Sciences
Tuomo Viitala
project engineer
Centria University of Applied Sciences
Antti Takalo
RD expert
Centria University of Applied Sciences
Tero Köyhäjoki
project engineer
Centria University of Applied Sciences
Egidija Rainosalo
RD coordinator
Centria University of Applied Sciences
tel. 044 725 0264

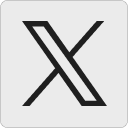

